Automobile technology sets the stage for a fascinating exploration of automotive innovation. From the evolution of the internal combustion engine to the rise of electric vehicles and autonomous driving, this field is constantly evolving, pushing the boundaries of engineering and design. This overview delves into the key advancements shaping the future of transportation, examining both the technological breakthroughs and the societal implications.
We will explore the intricacies of electric vehicle batteries, the challenges of creating safe autonomous driving systems, and the importance of vehicle connectivity and cybersecurity. Furthermore, we’ll discuss the role of lightweight materials, the impact of artificial intelligence, and the pursuit of sustainable manufacturing practices in the automotive industry. The future of automobiles promises even more exciting developments, and this exploration aims to provide a clear understanding of the current state and future trajectory of this dynamic field.
The Evolution of the Internal Combustion Engine
The internal combustion engine (ICE), a cornerstone of modern transportation, has undergone a dramatic transformation over the past century. From its relatively inefficient and polluting beginnings, it has evolved into a sophisticated piece of machinery, although still facing significant challenges in terms of fuel efficiency and emissions. This evolution is marked by continuous innovation in design, materials, and control systems.
The early 20th century saw the dominance of relatively simple, low-power engines. These were largely characterized by low compression ratios, inefficient carburetion, and rudimentary ignition systems. Technological advancements, however, steadily improved performance and reliability. The introduction of higher compression ratios, improved fuel injection systems (first carburetors, then fuel injection), and advanced ignition systems significantly boosted power output and fuel economy.
Engine Types and Efficiency
Different engine types offer varying levels of efficiency and power characteristics. Gasoline engines, prevalent in passenger vehicles, generally offer higher power output at lower engine speeds compared to diesel engines. However, diesel engines, typically used in heavier vehicles, are known for their higher fuel efficiency due to their higher compression ratios and the nature of diesel fuel combustion. The thermal efficiency of a gasoline engine typically ranges from 20% to 30%, while diesel engines can achieve efficiencies between 30% and 45%. Hybrid engines, combining an ICE with an electric motor, offer improved fuel efficiency by allowing the engine to operate at its optimal efficiency range and utilizing regenerative braking to recapture energy. The efficiency of a hybrid system varies depending on its design and driving conditions, but it generally surpasses that of comparable gasoline or diesel engines.
Challenges in ICE Development, Automobile technology
Developing more fuel-efficient and less polluting ICEs presents significant challenges. Stringent emission regulations necessitate the implementation of advanced aftertreatment systems such as catalytic converters and diesel particulate filters. These systems, while effective in reducing emissions, add complexity and cost. Further improvements in fuel efficiency require innovations in engine design, such as variable valve timing, downsizing (using smaller engines with turbocharging or supercharging), and advanced combustion strategies (e.g., homogeneous charge compression ignition – HCCI). However, optimizing these technologies for various operating conditions and maintaining durability remain significant hurdles. For example, while HCCI offers the potential for significantly higher efficiency, its control and stability under diverse operating conditions are still areas of active research. The development of alternative fuels, such as biofuels and synthetic fuels, also holds promise but requires significant infrastructure investment and further research to ensure their sustainability and environmental impact are minimized. The long-term viability of the ICE depends on overcoming these challenges and achieving significant reductions in both fuel consumption and greenhouse gas emissions.
Electric Vehicle Technology
Electric vehicles (EVs) represent a significant advancement in automotive technology, offering a cleaner and potentially more efficient alternative to internal combustion engine (ICE) vehicles. Their widespread adoption hinges on several key factors, including battery technology, charging infrastructure, and overall cost-effectiveness. This section delves into the intricacies of EV battery types and the associated charging infrastructure requirements.
Electric Vehicle Battery Types and Performance Characteristics
Several battery chemistries power electric vehicles, each with its strengths and weaknesses regarding energy density, lifespan, charging speed, and cost. Lithium-ion batteries currently dominate the EV market due to their high energy density and relatively long lifespan. However, different types of lithium-ion batteries exist, each with nuanced performance characteristics. These include Lithium Iron Phosphate (LFP), Nickel Manganese Cobalt (NMC), and Nickel Cobalt Aluminum (NCA) batteries.
LFP batteries, known for their safety and long cycle life, typically offer lower energy density compared to NMC and NCA batteries. This translates to a shorter driving range on a single charge. NMC and NCA batteries, on the other hand, boast higher energy density but may have a shorter lifespan and present slightly higher safety concerns. The choice of battery chemistry often depends on the vehicle’s intended use, desired range, and cost considerations. For instance, LFP batteries are frequently found in more budget-friendly EVs, while NMC and NCA batteries are more common in high-performance or long-range vehicles.
Charging Infrastructure Requirements for Electric Vehicles
The charging infrastructure for EVs varies significantly depending on the battery size and charging capabilities of the vehicle. Level 1 charging uses a standard household outlet and provides the slowest charging rate, suitable for overnight charging. Level 2 charging, utilizing a dedicated 240-volt outlet, offers a faster charging rate, typically suitable for home or workplace charging. Level 3, or DC fast charging, provides the fastest charging speeds, enabling significant battery replenishment within a relatively short time frame. However, this type of charging requires specialized and expensive equipment. The charging infrastructure needs differ greatly depending on the vehicle type and user requirements; a city commuter might rely primarily on Level 2 charging at home, while long-distance travelers would benefit significantly from a robust network of Level 3 fast-charging stations.
Hypothetical Charging Network for a Major Metropolitan Area
Designing a comprehensive charging network for a major metropolitan area requires careful consideration of several factors, including population density, traffic patterns, and accessibility. The following table Artikels a hypothetical charging network for a city similar in size to Chicago, Illinois, focusing on strategically placed charging stations to maximize accessibility and minimize range anxiety. This network integrates a mix of Level 2 and Level 3 charging stations to cater to various user needs.
Location | Charging Level | Number of Chargers | Accessibility Features |
---|---|---|---|
Downtown Core (multiple locations) | Level 2 & Level 3 | 50 (Level 2) & 20 (Level 3) per location | High pedestrian and vehicle traffic, close proximity to public transportation |
Major Residential Areas (distributed) | Level 2 | 10-20 per location | Residential parking lots, apartment complexes, community centers |
Major Highways and Arterial Roads (strategically placed) | Level 3 | 4-8 per location | Easy highway access, ample parking space, well-lit areas |
Shopping Malls and Commercial Centers | Level 2 & Level 3 | 20 (Level 2) & 10 (Level 3) per location | Convenient access for shoppers, ample parking, often with amenities |
Autonomous Driving Systems
Autonomous driving systems represent a significant leap forward in automotive technology, promising increased safety, efficiency, and accessibility. These systems rely on a complex interplay of sophisticated sensors, powerful computing, and advanced algorithms to perceive the environment, make decisions, and control the vehicle’s movement without human intervention. The development and deployment of these systems are complex and multifaceted, involving considerable engineering and ethical considerations.
Autonomous vehicles utilize a suite of sensor technologies to build a comprehensive understanding of their surroundings. This sensor fusion is crucial for robust and reliable operation in diverse and unpredictable environments.
Sensor Technologies in Autonomous Vehicles
The ability of an autonomous vehicle to navigate safely and effectively relies heavily on its capacity to accurately perceive its environment. This is achieved through a combination of different sensor technologies, each with its strengths and limitations. LiDAR, radar, and cameras are the primary sensor modalities, often working in concert to provide a robust and redundant perception system.
LiDAR (Light Detection and Ranging) systems emit laser beams to create a 3D point cloud representation of the surrounding environment. This detailed point cloud allows the vehicle to accurately measure distances and identify objects with high precision, even in low-light conditions. However, LiDAR systems can be expensive and their performance can be affected by adverse weather conditions like heavy fog or rain.
Radar (Radio Detection and Ranging) systems use radio waves to detect objects and measure their distance and velocity. Radar is less susceptible to adverse weather conditions than LiDAR and can penetrate fog and light rain, providing reliable data even in challenging environments. However, radar’s resolution is generally lower than LiDAR, resulting in less detailed object information.
Cameras provide visual information about the environment, allowing the vehicle to interpret traffic signs, lane markings, and the behavior of other road users. Cameras are relatively inexpensive and provide rich visual data, but their performance can be significantly affected by poor lighting conditions and adverse weather. Advanced computer vision algorithms are essential for processing the vast amounts of visual data captured by cameras.
Challenges in Developing Safe and Reliable Autonomous Driving Systems
Developing safe and reliable autonomous driving systems presents several significant challenges. The complexity of real-world driving scenarios, the unpredictable behavior of other road users, and the need for robust fault tolerance are key hurdles.
One major challenge lies in handling edge cases – unexpected or rare situations that are difficult to anticipate and program for. For example, a child unexpectedly running into the street or a sudden, unexpected obstacle appearing in the vehicle’s path requires the autonomous system to react quickly and safely. Ensuring the system’s ability to reliably handle these situations is a critical area of ongoing research and development.
Another challenge is the need for robust sensor fusion and data processing. The autonomous vehicle must integrate data from multiple sensors to create a consistent and accurate understanding of its environment. This requires sophisticated algorithms that can effectively combine data from different sources, handle inconsistencies, and account for sensor noise and errors.
Finally, ethical considerations are paramount. Programming autonomous vehicles to make decisions in unavoidable accident scenarios raises complex ethical questions. Defining clear guidelines and regulations for the behavior of autonomous vehicles in such situations is crucial for ensuring public trust and safety.
Societal Impacts of Widespread Autonomous Vehicle Adoption
The widespread adoption of autonomous vehicles could have profound societal impacts, affecting transportation, urban planning, and the economy.
Potential benefits include reduced traffic congestion, improved road safety (due to reduced human error), increased accessibility for people with disabilities, and enhanced efficiency in logistics and transportation networks. For example, autonomous delivery vehicles could significantly improve the speed and efficiency of package delivery, reducing costs and improving service.
However, there are also potential negative consequences. Job displacement in the transportation sector is a major concern, as autonomous vehicles could potentially replace millions of human drivers. Furthermore, questions about data privacy and security, as well as the potential for algorithmic bias, need careful consideration. The equitable distribution of the benefits of autonomous vehicle technology is also a critical societal issue that requires careful planning and policy-making.
Advanced Driver-Assistance Systems (ADAS)
Advanced Driver-Assistance Systems (ADAS) represent a significant leap forward in automotive safety and convenience. These systems utilize a variety of sensors, cameras, and radar to monitor the vehicle’s surroundings and assist the driver, ultimately aiming to reduce accidents and improve the overall driving experience. The effectiveness of these systems varies greatly depending on the specific technology employed and the prevailing driving conditions.
Common ADAS Features and Their Functionality
Several ADAS features are now commonplace in modern vehicles. These features offer varying degrees of driver assistance, ranging from simple alerts to more active interventions. Understanding their individual functions is crucial for safe and effective utilization.
Adaptive Cruise Control (ACC) automatically adjusts the vehicle’s speed to maintain a pre-set distance from the vehicle ahead. Using radar or lidar, ACC can accelerate or decelerate the car, even to a complete stop in some systems, resuming speed once the path is clear. Lane Keeping Assist (LKA) uses cameras to detect lane markings and gently steer the vehicle back into its lane if it begins to drift. Blind Spot Monitoring (BSM) uses sensors to detect vehicles in the driver’s blind spots, alerting them with visual or audible warnings. Automatic Emergency Braking (AEB) can automatically apply the brakes to avoid or mitigate a collision, often engaging at low speeds first. Forward Collision Warning (FCW) provides an alert to the driver when a potential collision is detected. Parking Assist systems use sensors and cameras to guide the driver into parking spaces, sometimes even taking over steering control.
Comparison of ADAS Effectiveness in Varying Conditions
The performance of ADAS features can be significantly impacted by environmental factors. For example, ACC may struggle in heavy traffic or poor visibility due to limitations in sensor range and accuracy. Similarly, LKA can be less effective on poorly marked roads or in inclement weather, where lane markings may be obscured. AEB systems generally perform well in clear conditions but may be less effective in scenarios involving pedestrians or cyclists, especially at higher speeds or in low-light conditions. The effectiveness of BSM can be reduced by the presence of large vehicles or obstructions that block the sensors’ view. Parking assist systems may struggle in tight or unusual parking spaces.
Hierarchical Structure of ADAS Features Based on Autonomy Level
The level of autonomy provided by different ADAS features varies considerably. A hierarchical structure can be used to categorize them based on their level of driver intervention required.
- Level 0: Driver Assistance: Features like FCW and BSM provide warnings but do not actively intervene in vehicle control. The driver remains fully responsible for all aspects of driving.
- Level 1: Driver Assistance: Systems like ACC and LKA offer partial automation of specific driving tasks, such as maintaining speed and lane position. The driver still retains ultimate control and must be prepared to take over at any time.
- Level 2: Partial Automation: Systems combining ACC and LKA, often with additional features like lane centering and traffic jam assist, provide more significant automation. However, the driver must still actively monitor the driving environment and be ready to intervene.
- Level 3: Conditional Automation: In limited conditions, the vehicle can control all aspects of driving. However, the driver must still be able to take over when prompted by the system. This level is currently less common.
Vehicle Connectivity and Telematics
The integration of telematics and connectivity systems has fundamentally reshaped the automotive landscape, transforming vehicles from isolated machines into intelligent, networked entities. This interconnectedness offers significant improvements in safety, maintenance, and the overall driving experience, paving the way for a more efficient and enjoyable transportation future. This section explores the multifaceted impact of vehicle connectivity and telematics.
Telematics in Enhancing Vehicle Safety and Maintenance
Telematics systems leverage various technologies, including GPS, cellular networks, and onboard sensors, to collect and transmit data about the vehicle’s performance and location. This data plays a crucial role in enhancing both safety and maintenance. Real-time location tracking, for instance, is vital for emergency response services, allowing for faster and more accurate dispatch of help in the event of an accident. Moreover, diagnostic trouble codes (DTCs) transmitted remotely can alert drivers and mechanics to potential issues before they escalate into major repairs, leading to proactive maintenance and preventing breakdowns. Predictive maintenance, based on the analysis of vehicle data, allows for scheduled servicing based on actual wear and tear rather than arbitrary mileage intervals, optimizing maintenance schedules and reducing downtime.
Enhanced Driver Experience Through Vehicle Connectivity
Vehicle connectivity dramatically enhances the driver experience beyond basic navigation and entertainment. Features such as remote vehicle diagnostics, allowing drivers to check their car’s health from their smartphone, offer convenience and peace of mind. Over-the-air software updates eliminate the need for physical visits to dealerships for software upgrades, ensuring the vehicle remains up-to-date with the latest features and security patches. Furthermore, connected infotainment systems provide seamless integration with smartphones and other smart devices, offering access to a wide range of applications and services, from music streaming to hands-free communication. The integration of personalized settings and driver profiles ensures a customized and comfortable driving experience.
Smart City Traffic Flow Optimization Using Vehicle Data
Imagine a city where traffic flow is optimized in real-time, reducing congestion and improving travel times. This vision is becoming a reality through the aggregation and analysis of data from connected vehicles. Data such as vehicle speed, location, and acceleration, collected from a large number of vehicles, can be used to identify traffic bottlenecks and congestion hotspots. This information can then be used to dynamically adjust traffic signals, reroute traffic, and provide drivers with real-time information on optimal routes. For example, a city might use aggregated data to detect a sudden slowdown on a major highway. The system could then adjust traffic light timings on intersecting streets to reduce the backup, potentially even diverting traffic to less congested alternate routes through notifications sent to drivers’ navigation systems. This data-driven approach can significantly improve traffic flow efficiency, reducing commute times and minimizing fuel consumption.
Lightweight Materials in Automotive Manufacturing: Automobile Technology
The automotive industry is under constant pressure to improve fuel efficiency and reduce emissions. A significant strategy in achieving these goals involves reducing vehicle weight. Lightweight materials, offering superior strength-to-weight ratios compared to traditional steel, are playing an increasingly crucial role in modern vehicle design and manufacturing. This section explores the use of these materials, their cost-effectiveness, and the manufacturing processes involved in their implementation.
The use of lightweight materials like aluminum and carbon fiber significantly reduces vehicle weight, leading to improved fuel economy. Lighter vehicles require less energy to accelerate and maintain speed, directly translating to lower fuel consumption and reduced CO2 emissions. Furthermore, reduced weight can enhance vehicle handling and performance, offering benefits beyond just fuel efficiency. The specific impact varies depending on the material used and the extent of its integration into the vehicle’s structure. For instance, replacing steel body panels with aluminum can result in a noticeable weight reduction, while employing carbon fiber reinforced polymers (CFRP) in critical structural components can yield even more substantial savings.
Cost-Effectiveness of Lightweight Materials
The cost-effectiveness of different lightweight materials varies considerably. Aluminum, while more expensive than steel, offers a good balance between cost and weight reduction benefits. Its widespread use in automotive manufacturing has led to economies of scale, making it a relatively accessible option for many manufacturers. Carbon fiber, on the other hand, is significantly more expensive than both steel and aluminum. Its high strength and stiffness make it ideal for high-performance vehicles and specific structural components, but the manufacturing process is complex and labor-intensive, driving up the overall cost. Magnesium alloys represent another option, offering a lighter alternative to aluminum, but their susceptibility to corrosion requires careful consideration in design and manufacturing. The ultimate choice depends on the specific application, performance requirements, and the manufacturer’s budget. A cost-benefit analysis, considering factors like material cost, manufacturing complexity, and potential fuel savings over the vehicle’s lifespan, is crucial in determining the optimal material selection.
Manufacturing Processes for Lightweight Materials
Incorporating lightweight materials into vehicle construction involves specialized manufacturing processes. Aluminum alloys are commonly used in body panels and chassis components through processes like casting, extrusion, and stamping. These techniques are relatively mature and well-established within the automotive industry. Carbon fiber, however, necessitates more complex procedures. The manufacturing of CFRP components often involves autoclave molding, a process where pre-preg carbon fiber sheets are placed in a mold and cured under high pressure and temperature. This method ensures high-quality, consistent components but is capital-intensive and requires skilled labor. Other techniques, such as resin transfer molding (RTM) and pultrusion, are also employed depending on the specific component geometry and desired properties. The choice of manufacturing process is influenced by factors such as component complexity, production volume, and desired mechanical properties. For example, high-volume production of simpler components might favor RTM, while complex, high-performance parts might require autoclave molding. The increasing adoption of lightweight materials necessitates significant investment in advanced manufacturing technologies and skilled workforce training.
Automotive Cybersecurity
The increasing connectivity of modern vehicles introduces significant cybersecurity vulnerabilities. These vulnerabilities, if exploited, can compromise vehicle functionality, data privacy, and even physical safety. Understanding these risks and implementing robust security measures is crucial for the continued development and adoption of connected car technologies.
Modern vehicles are essentially sophisticated computers on wheels, incorporating numerous interconnected systems controlled by embedded software. This complexity creates numerous attack surfaces, making them attractive targets for malicious actors. The consequences of successful cyberattacks range from minor inconveniences, such as remote unlocking or disabling of infotainment features, to far more serious consequences, including loss of vehicle control, data theft, and even physical harm.
Potential Cybersecurity Vulnerabilities in Modern Vehicles
Several entry points exist for cyberattacks against vehicles. These vulnerabilities can be broadly categorized into hardware, software, and network-related weaknesses. For instance, compromised Electronic Control Units (ECUs), vulnerabilities in telematics systems, and weaknesses in over-the-air (OTA) update mechanisms all represent significant risks. Furthermore, the increasing reliance on external network connections, such as cellular networks and Wi-Fi, expands the attack surface, allowing remote access to vehicle systems. An example is a vulnerability in a vehicle’s onboard diagnostic (OBD) port, which could allow an attacker to gain control of various vehicle functions.
Methods Used to Protect Vehicles from Cyberattacks
Protecting vehicles from cyberattacks requires a multi-layered approach. This involves incorporating robust security measures at various levels, from the hardware and software components to the network infrastructure. Examples include secure coding practices to minimize software vulnerabilities, encryption of sensitive data transmitted over the network, and regular software updates to patch known vulnerabilities. Hardware-level security measures, such as secure boot mechanisms and tamper-resistant ECUs, are also crucial. Furthermore, employing intrusion detection and prevention systems helps monitor vehicle networks for suspicious activity and block malicious attempts. The automotive industry is also actively developing advanced security technologies, such as blockchain-based solutions for secure data management and authentication.
A Comprehensive Cybersecurity Strategy for a Connected Vehicle
A comprehensive cybersecurity strategy for a connected vehicle should be proactive and encompass all stages of the vehicle lifecycle, from design and development to deployment and maintenance. This strategy must integrate various security controls and measures to mitigate the risks associated with vehicle connectivity. It should involve rigorous security testing throughout the development process, regular security audits to identify and address vulnerabilities, and a robust incident response plan to effectively manage security incidents. Furthermore, it is essential to establish clear security policies and procedures, providing guidelines for secure software development, network access control, and data protection. The strategy must also include provisions for secure over-the-air (OTA) updates to quickly deploy security patches and address newly discovered vulnerabilities. Finally, close collaboration between vehicle manufacturers, cybersecurity experts, and regulatory bodies is critical to ensure the ongoing security of connected vehicles.
The Impact of Artificial Intelligence on Automobile Technology
Artificial intelligence (AI) is rapidly transforming the automotive industry, moving beyond mere automation to create vehicles that are safer, more efficient, and more personalized. Its influence spans various aspects of automotive technology, from enhancing driver assistance features to enabling fully autonomous driving capabilities. This section will explore the multifaceted role of AI in modern and future automobiles, addressing both its benefits and the ethical considerations it raises.
AI’s applications in the automotive sector are extensive and continually evolving. Its core strength lies in its ability to process vast amounts of data and learn from it, enabling improvements in performance and decision-making that were previously impossible.
AI in Autonomous Driving
Autonomous driving relies heavily on AI algorithms to interpret sensor data (from cameras, lidar, radar, etc.), map environments, plan routes, and control vehicle actions. Deep learning models, for instance, are trained on massive datasets of driving scenarios to recognize objects, predict their movement, and make informed decisions about navigation and safety. Companies like Tesla, Waymo, and Cruise are actively developing and deploying AI-powered autonomous driving systems, although full autonomy remains a significant technological challenge. The success of these systems hinges on the ability of AI to handle unpredictable situations and ensure passenger safety. For example, AI must be capable of reliably distinguishing between a pedestrian and a moving object like a shopping cart to avoid accidents.
AI in Driver Assistance Systems
AI enhances existing driver assistance systems (ADAS) such as adaptive cruise control, lane keeping assist, and automatic emergency braking. AI algorithms can analyze driver behavior, road conditions, and surrounding traffic to provide more responsive and tailored assistance. For example, AI-powered adaptive cruise control can predict and adjust to the speed and braking of vehicles ahead more smoothly than traditional systems, improving both comfort and safety. Similarly, AI-enhanced lane keeping assist can better anticipate lane changes and provide more nuanced steering corrections.
AI in Predictive Maintenance
AI plays a crucial role in predictive maintenance, helping to anticipate potential vehicle failures before they occur. By analyzing data from various vehicle sensors (engine temperature, tire pressure, etc.), AI algorithms can identify patterns indicative of impending problems. This allows for proactive maintenance, minimizing downtime and reducing the risk of costly repairs or accidents. For example, an AI system might detect an anomaly in engine performance, predicting an impending failure several days in advance, giving the owner ample time for preventative maintenance. This proactive approach significantly improves vehicle reliability and operational efficiency.
Ethical Considerations of AI in Automobiles
The use of AI in automobiles raises several ethical concerns. One key issue is the allocation of responsibility in accidents involving autonomous vehicles. Determining liability when an AI system makes an error is a complex legal and ethical challenge. Another concern is the potential for bias in AI algorithms. If training data reflects existing societal biases, the AI system may perpetuate or even amplify these biases in its decision-making, leading to unfair or discriminatory outcomes. Data privacy is another critical consideration, as AI systems collect and process large amounts of personal data about drivers and their behavior. Ensuring the responsible and ethical use of this data is paramount. Transparency in AI algorithms is also crucial; understanding how these systems make decisions is essential for building public trust and addressing potential biases.
Future Applications of AI in the Automotive Industry
The future of AI in the automotive industry holds immense potential. Beyond autonomous driving and enhanced ADAS, AI could be used to personalize the driving experience, optimizing vehicle settings based on individual preferences and driving styles. AI could also improve traffic management, optimizing traffic flow and reducing congestion through intelligent coordination of autonomous vehicles. Furthermore, AI could facilitate the development of more sustainable and efficient vehicles, optimizing fuel consumption or energy usage through real-time adjustments based on driving conditions and vehicle status. The integration of AI into the automotive ecosystem promises a future of safer, more efficient, and more personalized transportation.
Sustainable Automotive Manufacturing Processes
The automotive industry, a significant contributor to global economic growth, also carries a substantial environmental burden. Traditional manufacturing processes are energy-intensive and generate considerable waste, impacting air and water quality, and contributing to climate change. The shift towards sustainable manufacturing is crucial not only for environmental protection but also for the long-term viability of the industry itself, increasingly influenced by stringent environmental regulations and growing consumer demand for eco-friendly products.
The environmental impact of traditional automotive manufacturing is multifaceted. From the extraction of raw materials like iron ore and bauxite to the energy-intensive processes of casting, forging, and machining, significant greenhouse gas emissions are generated. Furthermore, the use of volatile organic compounds (VOCs) in paints and coatings contributes to air pollution, while the disposal of manufacturing waste, including hazardous materials, poses environmental risks. Water consumption is also substantial, particularly in processes like cleaning and cooling. These combined factors highlight the urgent need for a more sustainable approach.
Environmentally Friendly Manufacturing Practices
Implementing environmentally friendly manufacturing practices requires a holistic approach across the entire production lifecycle. This includes optimizing energy efficiency through the adoption of renewable energy sources and improved process technologies. Waste reduction strategies, such as closed-loop systems for recycling materials and minimizing waste generation, are essential. Furthermore, the use of cleaner production technologies, such as water-based paints and coatings, reduces the emission of harmful pollutants. Investing in advanced manufacturing technologies, such as additive manufacturing (3D printing), can improve material utilization and reduce waste. Finally, robust environmental management systems are crucial to monitor and control emissions and waste throughout the production process. Companies are increasingly adopting lifecycle assessments (LCA) to comprehensively evaluate the environmental impact of their products and processes, allowing for targeted improvements.
Sustainable Materials Used in Automotive Production
The automotive industry is increasingly adopting sustainable materials to reduce its environmental footprint. The use of lightweight materials, such as aluminum and carbon fiber, reduces fuel consumption and emissions. Bio-based materials, derived from renewable sources like plants, are being explored as alternatives to traditional petroleum-based plastics. Recycled materials, including steel and aluminum scrap, are being increasingly incorporated into vehicle components, reducing the demand for virgin materials. For example, some manufacturers are using recycled aluminum in body panels, while others are integrating recycled plastics into interior components. The use of hemp fibers in composite materials offers another avenue for sustainable material integration, demonstrating the growing diversity of environmentally friendly options in automotive manufacturing. The development and application of these sustainable materials are vital steps towards creating a more environmentally responsible automotive industry.
The Future of Automobile Technology
The next 10-20 years promise a dramatic reshaping of the automotive landscape, driven by converging technological advancements. We are poised to see vehicles that are not only more efficient and safer but also seamlessly integrated into our digital lives and profoundly impacting urban planning and societal structures. These changes will be fueled by ongoing improvements in existing technologies and the emergence of entirely new paradigms.
The convergence of several key technological areas will define the future of automobiles. These advancements will lead to vehicles that are more personalized, sustainable, and interconnected than ever before.
Potential Breakthroughs in Automotive Technology
Several key areas are ripe for significant breakthroughs. Solid-state batteries, for example, promise significantly higher energy density and faster charging times compared to current lithium-ion batteries, leading to electric vehicles with extended ranges and reduced charging times, mirroring the rapid advancements seen in smartphone battery technology over the last decade. Further advancements in hydrogen fuel cell technology could also offer a compelling alternative, addressing range anxiety and refueling times. In autonomous driving, we can expect to see a move beyond Level 4 autonomy towards fully autonomous Level 5 systems, capable of handling all driving situations without human intervention. This will require significant improvements in sensor technology, artificial intelligence, and robust cybersecurity measures. Finally, advancements in materials science will continue to lead to lighter, stronger, and more sustainable vehicle components, further improving fuel efficiency and reducing emissions. The development of advanced bio-based materials offers a particularly exciting prospect for reducing the environmental impact of automotive manufacturing.
Long-Term Societal Impact of Technological Advancements
The widespread adoption of autonomous vehicles has the potential to revolutionize transportation systems. Reduced congestion, improved traffic flow, and enhanced safety are all potential benefits. The shift to electric vehicles, coupled with sustainable manufacturing processes, will significantly reduce greenhouse gas emissions and improve air quality, particularly in urban areas. This transition will, however, require substantial investment in charging infrastructure and the responsible sourcing of raw materials for batteries. Furthermore, the increasing connectivity of vehicles will lead to the collection of vast amounts of data, raising important questions about data privacy and security. The potential for job displacement in the automotive industry due to automation also necessitates proactive measures to reskill and upskill the workforce. The integration of advanced driver-assistance systems (ADAS) is already improving road safety, and this trend is expected to continue with the advancement of autonomous driving technology.
A Futuristic Vehicle: The “Synapse”
Imagine a sleek, aerodynamic vehicle, the “Synapse,” constructed from lightweight, bio-based composite materials. Its exterior is a shimmering, self-healing surface that adapts to changing weather conditions. The Synapse is fully autonomous, navigating seamlessly through traffic using advanced sensor fusion technology and AI-powered decision-making. Its interior is a minimalist, personalized space featuring haptic controls and holographic displays, adapting to the driver’s preferences and needs. The vehicle is powered by a solid-state battery offering a range exceeding 1000 miles on a single charge, with wireless charging capabilities. The Synapse is seamlessly integrated into a smart city ecosystem, providing real-time traffic information, parking assistance, and personalized routing optimized for energy efficiency and travel time. The vehicle’s exterior seamlessly integrates solar panels to contribute to its energy needs, further emphasizing its sustainable design. The Synapse represents a glimpse into a future where personal transportation is efficient, safe, sustainable, and deeply integrated into the fabric of our lives.
Human-Machine Interface (HMI) Design in Vehicles
The human-machine interface (HMI) in automobiles has undergone a dramatic transformation, evolving from simple analog gauges and basic controls to sophisticated, integrated systems incorporating touchscreens, voice commands, and even gesture recognition. This evolution reflects advancements in computing power, display technology, and our understanding of human-computer interaction within the demanding context of driving. The goal remains consistent: to provide drivers with the information and control they need, safely and intuitively, minimizing distraction and maximizing situational awareness.
The design of automotive HMIs is a complex process balancing functionality, safety, and aesthetics. The choice of interface significantly impacts the driver experience, influencing factors like comfort, ease of use, and ultimately, safety on the road. Different approaches cater to various preferences and driving scenarios.
A Comparison of HMI Approaches
Touchscreens have become ubiquitous in modern vehicles, offering a large display area for information and controls. However, their use while driving can be distracting, particularly for complex tasks. Voice control systems offer a hands-free alternative, allowing drivers to interact with the vehicle without taking their eyes off the road. However, voice recognition technology is still prone to errors, especially in noisy environments. Gesture control, while still relatively uncommon, offers a potentially more intuitive and less distracting way to interact with vehicle systems, but its implementation requires sophisticated sensors and algorithms. Each approach presents advantages and disadvantages, leading to hybrid systems combining multiple interaction modalities.
Designing a Futuristic Vehicle User Interface
A futuristic vehicle HMI should prioritize safety and intuitive operation, seamlessly integrating information and controls to minimize driver workload and enhance the driving experience. The design should anticipate advancements in autonomous driving technology and integrate seamlessly with advanced driver-assistance systems (ADAS).
- Holistic Display System: A curved, high-resolution display encompassing the entire dashboard, providing a panoramic view of vehicle information, navigation, entertainment, and ADAS alerts. The display would dynamically adapt its layout and content based on the driving context and the driver’s preferences. For example, in autonomous driving mode, the display could transition to a more relaxed mode, offering entertainment options or work functionalities.
- Contextual Information Prioritization: The system would prioritize critical information based on the driving situation. For instance, during emergency braking, all other information would be suppressed to highlight crucial safety alerts. This prioritization would leverage advanced algorithms and sensor data to dynamically adjust the display’s content.
- Predictive and Proactive Interface: The HMI would anticipate the driver’s needs and proactively offer relevant information or controls. For example, if the system detects low fuel, it would proactively suggest nearby gas stations. Similarly, if the navigation system detects potential traffic congestion, it would proactively suggest alternative routes.
- Biometric Monitoring and Personalized Adaptation: The system would integrate biometric sensors to monitor the driver’s physiological state, such as heart rate and stress levels. This information would be used to personalize the HMI’s responsiveness and provide appropriate alerts or suggestions. For instance, if the system detects signs of driver fatigue, it might suggest taking a break or adjusting the cabin environment.
- Seamless Integration with Autonomous Driving: In autonomous mode, the HMI would transition to a more relaxed and informative interface, offering options for entertainment, communication, or work-related tasks. The transition would be smooth and intuitive, clearly indicating the driving mode and providing appropriate feedback.
Final Review
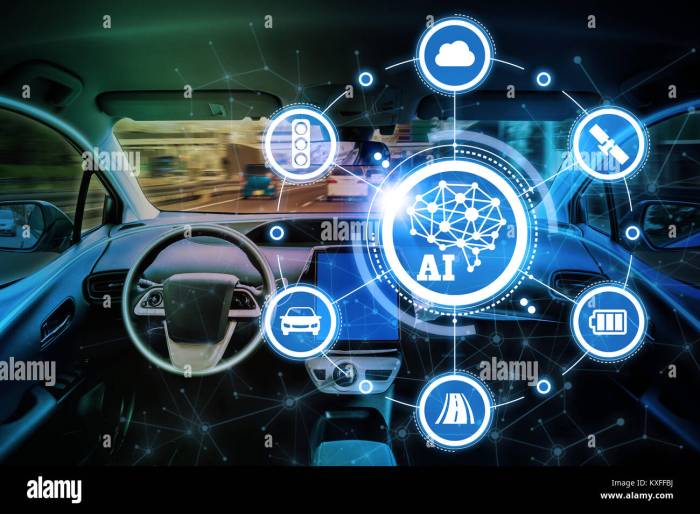
The automotive industry is undergoing a period of unprecedented transformation, driven by technological advancements and a growing focus on sustainability. From the internal combustion engine’s refinement to the emergence of fully autonomous vehicles, the journey has been remarkable. The integration of artificial intelligence, enhanced connectivity, and a commitment to eco-friendly manufacturing practices are shaping a future where vehicles are safer, more efficient, and better integrated into our smart cities. Understanding these advancements is crucial to navigating the evolving landscape of personal and public transportation.